Thank you for visiting nature.com. You are using a browser version with limited support for CSS. To obtain the best experience, we recommend you use a more up to date browser (or turn off compatibility mode in Internet Explorer). In the meantime, to ensure continued support, we are displaying the site without styles and JavaScript.
Scientific Reports volume 13, Article number: 6888 (2023 ) Cite this article 12-dion

Radix Astragali is one of the most famous and frequently used health food supplements and herbal medicines. Among more than 227 components of Radix Astragali, Astragaloside IV (AG IV) is famous functional compound and is commonly used as a quality marker for Radix Astragali. However, the relatively low content of AG IV in Radix Astragali (< 0.04%, w/w) severely limits its application. The purpose of this study is to improve the biotransformation of AG IV and its bioaccessibility during in vitro digestion by Poria cocos solid fermenting Radix Astragali. The optimum fermentation conditions were as follows: Inoculation amount 8 mL; fermentation time 10 d; fermentation humidity 90%. Through fermentation, the content of AG IV was increased from 384.73 to 1986.49 μg/g by 5.16-fold. After in vitro digestion, the contents of genistin, calycosin, formononetin, AG IV, Astragaloside II (AG II) and total flavonoids in fermented Radix Astragali (FRA) of enteric phase II (ENTII) were 34.52 μg/g, 207.32 μg/g, 56.76 μg/g, 2331.46 μg/g, 788.31 μg/g, 3.37 mg/g, which were 2.08-fold, 2.51-fold, 1.05-fold, 8.62-fold, 3.22-fold and 1.50-fold higher than those of control, respectively. The Scanning electron microscopy (SEM) of FRA showed rough surface and porous structure. The DPPH and ABTS radical scavenging rate of FRA were higher than those of control. These results showed that the Poria cocos solid fermentation could increase the content of the AG IV in Radix Astragali and improve the bioaccessibility and antioxidant activity of Radix Astragali, which is providing new ideas for future development and utilization of Radix Astragali.
Radix Astragali is the dried root of Astragalus membranaceus (Fisch.) Bge. var. mongholicus (Bge.) Hsiao or A. membranaceus (Fisch.) Bge. in the leguminous family. It is a famous traditional medicine and healthy food supplement in China with the beneficial effects for human health1. Furthermore, it is widely applied as a health additive in foods, teas, drinks, wines and so on. Radix Astragali is abundant with biologically active compounds, such as astragalosides, flavonoids, polysaccharide and various trace elements2. Among them, AG IV is the most significant compound for the pharmacological activities and therapeutic efficacy. Modern pharmacological studies showed that it has good effects on the cardiovascular system, immune, digestive, nervous system. There were many therapeutic effects for AG IV, such as anti-inflammatory, anti-fibrotic, anti-oxidative stress, anti-asthma, anti-diabetes, immunoregulation and cardioprotective effect3,4. However, the extraction rate of AG IV in Radix Astragali is limited by the conventional production technology. And there are lots of lignin, cellulose and pectin tightly bound to bioactive compounds in Radix Astragali and these substances inhibit the release of bioactive compounds into the digestive system. Thus, in order to solve this problem, some studies are devoted to microbe ferment Radix Astragali. The microbial fermentation can produce multienzyme system, which can transform the other astragalosides to AG IV5. On the other hand, the enzymes can hydrolyze the lignin, cellulose and pectin of Radix Astragali6, which can promote the release of the AG IV.
Poria cocos is the dried sclerotium of Poria cocos (Schw.)Wolf., which is an important edible and medicinal fungus. It has a long history of medicinal use in China and other Asian countries7. Research has demonstrated that Poria cocos have biological activities described as adjusting the immune function, antitumor and anti-inflammatory, etc8. In recent decades, the fermentation culture of Poria cocos has been developed because of the potential for the increased production of mycelia and bioactive components in a compact space and in a shorter period. During the fermentation process, Poria cocos makes full use of cellulose and other components as a carbon source to degrade the cell wall of the plant, which is contribute to the release of the bioactive compounds of the plant. In addition, as a consequence of its nutritional and health values, it is also applied as functional food. The combined use of Radix Astragali and Poria cocos has a long history, with the effect of tonifying the spleen and kidney. So far, there are no reports about fermentation of Radix Astragali using Poria cocos.
Currently, in vitro digestion techniques are commonly used to study the bioaccessibility of herbs and foods in order to better investigate the physicochemical changes and metabolism of functionally active substances during digestion. The microbial fermentation can promote the release of bioactive compounds into the digestive system. In order to investigate the bioaccessibility and digestion of Radix Astragali before and after fermentation, in vitro digestion experiments were conducted in the study. In vitro digestion system is an in vitro model for predicting or evaluating the digestibility, bioaccessibility, release kinetic characteristics and structural changes of compounds based on human gastrointestinal tract physiological processes and simulating in vivo digestion and absorption under in vitro conditions. The system can completely or partially replace in vivo tests and has the advantages of cost savings, shorter test cycles, accuracy and manual monitoring.
In this study, Radix Astragali was fermented by Poria cocos and used in vitro digestion and antioxidant technique to investigate the bioaccessibility and antioxidant activity before and after fungus fermentation, which provided a new idea for the future research of Radix Astragali.
A time-course study was performed on mass accumulation of Poria cocos cultured in 100 mL of 26.1 g/L potato liquid medium. The kinetics of the growth curves of Poria cocos was determined initially (Fig. 1). From 2 to 6 days, the dry weight of mycelium increased rapidly, which is the logarithmic phase of Poria cocos. On the 6th day, the growth trend of Poria cocos tends to stabilize. The peaks occurred at 7 d . Beyond 7 d, the culture entered a senescence phase. At the 7th day, the culture achieved the maximal mass accumulation and the production of secondary metabolites reached its maximum. According to the growth curve of Poria cocos to determine the incubation time of Poria cocos9.
Growth curves of Poria cocos.
Flavonoids and saponins are the basic active ingredients of plant extracts. The contents of flavonoids and saponins compounds present in fermentation Radix Astragali extract was determined by spectrophotometer and HPLC. After fermentation, the contents of AG IV and genistein increased. The factors concerning Poria cocos solid fermented Radix Astragali include inoculation amount, fermentation time and fermentation humidity. The influence of each factor was studied by single-factor experiments. All assays were conducted in triplicate.
The effect of inoculation amount on fermentation was studied under the conditions of fermentation temperature of 27 °C, fermentation time of 7 d, fermentation humidity of 90%. The inoculation amount ranged from 4 to 9 mL for the single-factor optimization test (Table 1). After fermentation, the contents of flavonoids, saponins and other active components in Radix Astragali showed different trends. The contents of calycosin-7-O-β-d -glucopyranoside (calycosin-glu), ononin, calycosin and formononetin were reduced significantly. The content of total saponins changed little and the content of total flavonoids increased. As the inoculation amount increased from 4 to 8 mL, the contents of genistein and AG IV increased. When the inoculation amount exceeded 8 mL, the contents of both compounds dereased. The reduction in the contents of calycosin-glu and other components may be due to hydrolytic enzymes produced by Poria cocos. These components were further converted to other secondary metabolite or smaller molecules10. The Poria cocos in growth and reproduction process may produce some enzymes, which can degrade cellulose, pectin and other substances in the cell wall of Radix Astragali and facilitate the release of chemical components. The increase of AG IV content may be due to the metabolism or biotransformation of other astragalosides as precursors in the fermentation process11. With the increase of inoculation amount, the contents of AG IV and other components decreased. Therefore, 8 mL of seed fermentation broth was chosen as the optimal inoculation amount.
The effect of fermentation time was studied under the conditions of fermentation temperature of 27 °C, inoculation amount of 8 mL, fermentation humidity of 90%. The fermentation time ranged from 6 d-11 d for the single-factor optimization experiment (Table 2). The changes of the contents of flavonoids, saponins and other active ingredients in Radix Astragali after fermentation were fluctuated. The contents of calycosin-glu, ononin, calycosin, formononetin, and AG II were significantly reduced after fermentation. The content of total saponins was lower than that of control and the content of total flavonoids increased. With the increase of fermentation days, the contents of genistin and AG IV increased first and then decreased. The maximum content of genistin was 34.75 μg/g on the 7th day and the maximum content of AG IV was 775.79 μg/g on the 10th day of fermentation. Poria cocos grew and metabolized vigorously in the early stage of fermentation. However, with the increase of fermentation days, the strain gradually aging, nutrient consumption increased, and metabolites reduced12. At 10 days, the mass accumulation of the content of AG IV achieved the maximum, so 10 days was chosen as the optimal fermentation time.
The fermentation humidity was investigated using different humidity-e.g., 65%, 90%, 115%, 140% and 165%-under the conditions of fermentation temperature of 27 °C, inoculation amount of 8 mL, fermentation time of 10 d (Table 3). With the increase of humidity, the contents of AG IV, AG II and total flavonoids increased and then decreased, and the content of genistin increased significantly. The highest content of genistein was 48.65 μg/g at the humidity of 165% and the highest content of AG IV was 1986.49 μg/g at the humidity of 90%. The Table 3 showed that with the increase of humidity from 65 to 90%, the content of AG IV increased gradually. However, with the increase of humidity to 165%, the content of AG IV reduced. Maybe the increase in fermentation humidity reduced the density of strains, released the contact inhibition of Poria cocos, which helped Poria cocos growth and metabolism. However, too much water caused the residue of Radix Astragali to produce stickiness, which produced a poor permeability and less dissolved oxygen environment for Poria cocos. It was not conducive to the growth and metabolism of Poria cocos. Because the content of AG IV increased the most when the humidity was 90%, 90% was chosen as the optimal fermentation humidity.
In the single-factor fermentation experiments, the changes of the contents of flavonoids, saponins and other active ingredients were fluctuated. Combined with the growth curves of Poria cocos (Fig. 1), the changes of the contents may be due to the growth of Poria cocos, which influnced the biotransformation of Radix Astragali. The fermentation conditions caused great fluctuations in the content of AG II. This phenomenon may be caused by the biotransformation of saponins, the other saponins first converted to AG II and then converted to AG IV13.
A thorough analysis of the tabular data showed the content of AG IV of the maximum factor for the optimal conditions. Meanwhile, AG IV is officially used as a quality-marker for Radix Astragali in Chinese Pharmacopoeia. The optimum fermentation conditions of the fermentation were as follows: 20 g of Radix Astragali, 90% humidity and 8 mL of inoculation amount were added and fermented at 27 °C for 10 days. In the optimum fermentation conditions, the content of AG IV was 1986.49 μg/g, which was 5.16 times higher than the control.
Bioaccessibility can be defined as the quantity which is released from the food matrix in the gastrointestinal tract and becomes available for absorption. However, it is difficult to study the in vivo changes of these components during their passage through the digestive tract. The in vitro measurement of bioaccessibility is now considered to be a reliable way to assess the availability of dietary compounds. The in vitro simulated gastrointestinal method consists of four phases: oral phase (OP), gastric phase (GP), enteric phase I (ENTI) and ENTII.
Table 4 shows the effects of in vitro digestion on the contents changes of main effective components in Radix Astragali before and after fermentation. The contents of genistin, calycosin, formononetin, AG IV, AG II in the FRA increased significantly, and the contents of each component in ENTII were 34.52, 207.32, 56.76, 2331.46 and 788.31 μg/g, which were 2.08-fold, 2.51-fold, 1.05-fold, 8.62-fold and 3.22-fold higher than those of the control, respectively. This is due to solid-state fermentation led to the destruction of the cell wall of Radix Astragali, promoting the release of flavonoids and saponins. On the other hand, gastrointestinal digestion significantly enhanced the release of effective components of Radix Astragali. Due to the action of the gastrointestinal digestive enzymes, the cellular structure of Radix Astragali and chemical bond of acetyl group and glycosidic were destroyed, and combined flavonoids and saponins were released14. The contents of calycosin glucoside and ononin decreased significantly.
In OP and GP, the contents of flavonoids and saponins of control and FRA had little change. After undergoing successive digestion in ENTI and ENTII, the contents of flavonoids and saponins increased, especially the total saponins increased significantly. The reason might be due to the fact that flavonoid and saponin components were in an acidic environment (during digestion in the stomach), which could suppress the release of them, while digestive enzymes in the intestine could hydrolyze proteins to release the protein-encapsulated flavonoids or saponins and they can exist stably in the weakly acidic environment15.
Bioaccessibility data of FRA and control are displayed in Table 4. The bioaccessibility of calycosin, formononetin, AG IV, AG II, total saponins and total flavonoids in the FRA were 274.34%, 260.61%, 117.36%, 83.63%, 437.83% and 125.28% and those of the control were 53.37%, 90.48%, 70.29%, 45.30%, 245.82% and 96.98%, respectively. The bioaccessibility of these components in the FRA were higher than those of the control. However, the bioaccessibility of calycosin-glu, genistin and ononin in the FRA were lower than those of the control. These results are possibly due to the glucosides have lots of oxhydryl, which are tightly bound to cellulose. After fermentation, the Poria cocos produced enzymes to hydrolyze cellulose, which can release the glucosides. And the multienzyme system produced by Poria cocos could promote the biotransformation of glucosides. On the other hand, the digestive enzymes could convert glucosides to aglycones during the in vitro digestion16. Thus the bioaccessibility of aglycones in the FRA were higher than those of control and the bioaccessibility of glucosides in the FRA were lower than those of control.
It is an interesting phenomenon that the contents of some bioactive components of control and FRA in fermentation experiment were higher than those in the vitro digestion experiment. For example, the content of calycosin in the control was 155.05 μg/g in Table 3 and 41.08 μg/g in Table 4 of OP. The content of calycosin in FRA was 75.57 μg/g in Table 3 and 62.34 μg/g in Table 4 of OP. On the one hand, it may be due to the solubility of the bioactive components in ethanol and digestion solution was different. On the other hand, the content of calycosin in FRA was higher than that of control in OP because the Poria cocos solid fermented Radix Astragali could promote the release of bioactive components. From this it can be seen that the Poria cocos solid fermented Radix Astragali could improve the bioaccessibility of Radix Astragali. In brief, in vitro digestion experiments validates that the FRA has higher bioaccessibility than control, which provides valuable information for FRA at different digestion stages.
The samples were examined by EVO LS15 SEM at a magnification of 2000x, in order to study the structural alterations of the Radix Astragali by fermentation and in vitro digestion. Figure 2 shows the control (A), the control in the gastric digestion (B), the control in the enteric II digestion (C), the FRA (D), the FRA in the gastric digestion (E), and the FRA in the enteric II digestion (F). The surface of control was smooth, basically no holes and with a small amount of particulate matter attached. Figure 2A indicated that the cells of control were not obviously destroyed and still retained the intact tissue structure. After fermentation, the microstructures were disorganized in Fig. 2D. The surface of FRA residues was rough, porous and reticular structure. These results may be due to Poria cocos mycelium could directly enter the drug residue and destroy the internal cells of Radix Astragali.
Scanning electron micrographs of each sample of Radix Astragali ((A) the control, (B) the control in the gastric digestion, (C) the control in the enteric II digestion, (D) the FRA, (E) the FRA in the gastric digestion, (F) the FRA in the enteric II digestion).
After gastric digestion, the electron micrographs of both samples changed significantly. Under the action of pepsin and acid digestion fluid, the sample was severely damaged. The surface became uneven and many particles were observed. As can be seen from Fig. 2B, E the fermentation samples were destroyed more seriously than the control samples. The results may be related to the destruction of Radix Astragali by Poria cocos. After fermentation, the surface of Radix Astragali showed a porous reticular structure, which increased the exposure area and made the active ingredients easier to digest and dissolve. After enteric II digestion, both samples were strongly destroyed, and the electron micrographs of Fig. 2C,F were not significantly different from those of Fig. 2B,E.
The various components have antioxidant activity in Radix Astragali. In the fermentation and in vitro digestion, the contents of AG IV, AG II, calycosin and genistin showed significant changes, thus their standards were selected as the reference for the antioxidant experiments of Radix Astragali. Here, the antioxidant capacities of ascorbic acid (Vc), non-fermented Radix Astragali extracts (control), FRA extracts and their in vitro digestive products were assessed with a DPPH radical scavenging assay and ABTS radical scavenging assay.
The DPPH antioxidant results of extracts and in vitro digested samples of Radix Astragali are shown in Fig. 3. The IC50 value of FRA was 0.140 mg/mL, which was higher than the IC50 value of the reference Vc (0.002 mg/mL) and calycosin (0.034 mg/mL), but superior to that of control (0.367 mg/mL). At 0.4 mg/mL, the DPPH free radical scavenging rate of the sample from high to low were: Vc 98.25%, calycosin 87.86%, FRA 63.81%, control 51.43%, FRA (OP) 35.59%, FRA (ENTII) 35.50%, FRA (GP) 34.52%, control (OP) 31.96%, FRA (ENTI) 31.94%, control (GP) 29.81%, genistin 24.52%, control (ENTII) 24.72%, control (ENTI) 20.42%, AGII 8.81% and AG IV 7.30%.
DPPH radical scavenging rate of each sample of Radix Astragali, standards and Vc.
The ABTS antioxidant results of extracts and in vitro digested samples of Radix Astragali are shown in Fig. 4. The IC50 value of FRA extracts was 0.064 mg/mL, which was higher than the IC50 value of the reference Vc (0.002 mg/mL), calycosin (0.003 mg/mL) and genistin (0.030 mg/mL) but superior to that of control (0.207 mg/mL). In the range of 0.01–0.30 mg/mL, the antioxidant capacity of the sample increased with the increase of mass concentration. At 0.29 mg/mL, the ABTS free radical scavenging rate of the sample from high to low were: Vc 99.86%, calycosin 99.28%, FRA 98.60%, genistin 87.26%, FRA (OP) 71.39%, control 68.72%, FRA (ENTI) 65.86%, FRA (ENTII) 64.73%, FRA (GP) 61.76%, control (OP) 46.44%, control (ENTII) 45.73%, control (ENTI) 45.30%, control (GP) 38.46%, AG II 11.14% and AG IV 7.09%.
ABTS radical scavenging rate of each sample of Radix Astragali, standards and Vc.
After fermentation and in vitro digestion, the DPPH and ABTS antioxidant capacities of FRA were all higher than that of control. However, the antioxidant activity of FRA and control decreased after in vitro digestion, which may be due to the weight of the extractum from the digestive fluid was higher than that of the FRA and control. Under the same dry mass concentration, the contents of antioxidant components in the antioxidant samples decreased, which affected the antioxidant capacity.
In conclusion, a scheme of solid fermentation of Radix Astragali with Poria cocos was proposed to increase the contents of the main active compound in Radix Astragali (AG IV). By optimizing the fermentation process, the best fermentation conditions are obtained: inoculation amount 8 mL; fermentation time 10 days; fermentation humidity 90%. Under optimal conditions, the content of AG IV increased from 384.73 to 1986.49 μg/g by 5.16-fold. Moreover, the bioaccessibility of FRA was investigated by in vitro digestion. After in vitro digestion, the contents of genistin, calycosin, formononetin, AG IV, AG II and total flavonoids of FRA in ENTII were 34.52 μg/g, 207.32 μg/g, 56.76 μg/g, 2331.46 μg/g, 788.31 μg/g, 3.37 mg/g, which were 2.08-fold, 2.51-fold, 1.05-fold, 8.62-fold, 3.22-fold and 1.50-fold higher than those of the control, respectively. And the bioaccessibility of calycosin, formononetin, AG IV, AG II, total saponins and total flavonoids of the FRA were 274.34%, 260.61%, 117.36%, 83.63%, 437.83% and 125.28% and those of the control were 53.37%, 90.48%, 70.29%, 45.30%, 245.82% and 96.98%, respectively. As observed through SEM, the surface of FRA and its in vitro digestion residue was rough and porous, appeared clearer reticular structure, and the surface area increased than control. In the DPPH and ABTS radical scavenging assay, the IC50 value of FRA was 0.140 mg/mL and 0.064 mg/mL, which were superior to that of control (0.367 mg/mL, 0.207 mg/mL). These results showed that the antioxidant of FRA was higher than that of control. After in vitro digestion, the FRA still have good antioxidant activity. In this study, Poria cocos was used to ferment Radix Astragali for the first time to complete the biotransformation of bioactive components. The FRA has high bioavailability and strong antioxidant activity, which provided a basis for the development and utilization of Radix Astragali.
Radix Astragali, the dried root of Astragalus membranaceus (Fisch.) Bge. var. mongholicus (Bge.) Hsiao was purchased from Shanxi Hunyuan Wansheng Huangqi Development Co., Ltd (Shanxi, China) and authenticated by Prof. Ying Lin at Binzhou Medical University (Yantai, Shandong). Voucher specimens were deposited in the herbarium of the Experimental Center of Chinese Medicine of Binzhou Medical University and the accession number was 2021–1003. Radix astragali was pulverized by a 800A disintegrator (Yongkang Hardware and Medical Instrument Plant, China) and passed through mesh sieve to obtain a fine powder with a particle size of 600 μm. Poria cocos (bio-08656) was purchased from Beijing Bai Ou Bo Wei Biotechnology Co., Ltd. (Beijing, China). Formic acid, methanol and acetonitrile of chromatographic grade were purchased from Tianjin Kemiou Chemical Reagent Co., Ltd. (Tianjin, China). Ultrapure water used in the whole experiment was purified by a Milli-Q water purification system from Millipore (Bedford, MA, USA). PDA medium and potato liquid medium were purchased from Qingdao Haibo Biotechnology Co., Ltd. (Qingdao, China). Potassium phosphate, potassium dihydrogen phosphate, sodium carbonate, potassium persulfate and sodium hydroxide were purchased from Sinopharm Chemical Reagent Co., Ltd. (Shanghai, China). Salivary amylase (BR, 4000u/g), pepsin (USP grade, 1:3000), gastric lipase (BR, 30,000u/g), pancreatic enzyme (BR, 1600u/g) and pig bile powder (BR) were purchased from Shanghai Yuanye biotech Co. (Shanghai, China). 1,1-diphenyl-2-picrylhydrazyl and 2,2-hydrazine-bis(3- ethylbenzothiazoline-6-sulfonic acid) diamine salt were purchased from Shanghai Macklin Biochemical Technology Co., Ltd. (Shanghai, China). Sodium nitrite was purchased from Tianjin Bodi Chemical Co., Ltd. (Tianjin, China). Aluminum nitrate was purchased from Shanghai walkai Biotechnology Co., Ltd. (Shanghai, China), Anhydrous ethanol was purchased from Tianjin Yongda Chemical Reagent Co., Ltd. (Tianjin, China). Vanillin was purchased from Tianjin Guangfu Fine Chemical Research Institute. Rutin (≥ 98%), Ononin (≥ 98%), Genistin (≥ 98%), Calycosin (≥ 98%), Calycosin-glu (≥ 99%), Formononetin (≥ 99%), AG II (≥ 98%) and AG IV (≥ 98%) were purchased from Chengdu Pusi biotech Co. (Chengdu, China).
All methods in this study were carried out in accordance with the relevant guidelines and regulations of the Pharmacopoeia of the People's Republic of China.
Poria cocos was inoculated on the culture dish with 46.0 g/L PDA medium, until the mycelium spread all over the culture dish. Then, the inoculated mycelium was cultured in 100 mL of 26.1 g/L potato liquid medium on a ZQPW-70 shaker incubator (Tianjin Laboratory Instrument Equipment Co., Ltd, Tianjin, China) at 27 °C, 180 rpm for 2 days–10 days. Following incubation, the mycelia were washed with water during filtration. Samples were placed in an oven at 60 ℃ until constant weight, and then the dry weight of the mycelia was measured. Each group had three parallel samples.
Twenty grams of Radix Astragali powder and 5 mL-25 mL ultrapure water were added to the fermentation bags. The mixture was put in an YXQ-Sll autoclave (Shanghai Boxun Medical Biological Instrument Co., Ltd, Shanghai, China) at 121 °C for 25 min. After that, 4 mL–9 mL of seed fermentation broth was inoculated on the medicinal powder of fermenting bag. Then, the mixture was incubated in a GSP-9160MBE constant temperature and humidity incubator (Shanghai Boxun Medical Biological Instrument) at 27 °C for 6 days–11 days. Each group had three parallel samples. The photo of product of Poria cocos fermented Radix Astragali was shown in Fig. 5A. Control experiments were Radix Astragali powder only with autoclaved treatment without Poria cocos. And ultrapure water replaced the seed fermentation broth to maintain the same moisture level.
Photo of Poria cocos fermented Radix Astragali ((A) the fermented Radix Astragali, (B) the small pieces of FRA, (C) the powder of FRA).
The in vitro simulated gastrointestinal method consists of four phases: oral phase OP, GP, ENTI and ENTII.
The FRA (Fig. 5A) was broken into small pieces (Fig. 5B) and dried at 60 °C. The dried samples were grounded into powders using a disintegrator and were passed through a 60-mesh sieve (Fig. 5C).
Five grams of the fermented sample and control were added into different conical bottle after sterilization. Ten milliliter 20 m mol/L phosphoric acid buffer was added, 0.9% normal saline was added to 125 mL, pH value was adjusted to 7.0 with 1 mol/L NaOH solution and kept at 37 °C for 10 min. Finally, 0.2 mg salivary amylase was added, mixed evenly. And put into a constant temperature water bath oscillator preheated at 37 °C, 55 rpm for 2 min in the dark. Four groups were digested at the same time. After digestion, they were heated to boiling in water for 2 min. One group was stored and the remaining 3 groups were continued for the next stage of digestion. Three parallel samples for each group.
After oral digestion, the remaining three groups of digestion samples were added to 125 mL with 0.9% normal saline. The pH value was adjusted to 2.0 with 1 mol/L HCl solution after mixing, and kept for 10 min at 37 °C in constant temperature water bath oscillator. Then, 0.375 g pepsin and 0.1125 mg lipase were added and mixed well. And put into a constant temperature water bath oscillator preheated at 37 °C, 150 rpm, for 2 h in the dark. After digestion, they were heated to boiling in water for 2 min. One group was stored and the remaining two groups were continued for the next stage of digestion.
Referring to the research of Ningtyas, et al.14, the enteric digestion phase was divided into two phases: ENTI and ENTII.
After gastric digestion, the remaining two groups of digestion samples were added to 125 mL with 0.9% normal saline. The pH value was adjusted to 4.7 with 1 mol/L NaOH solution, and the sample was kept for 10 min at 37 °C. Then, 0.125 g trypsin and 1.25 g bile were added and mixed well. The enteric I stage was digested for 2 h at 37 °C for 150 rpm in a water bath oscillator in the dark condition. After digestion, they were heated to boiling in water for 2 min. One group was stored and the other was continued for the next stage of digestion.
The last group of digested sample was added to 125 mL by the same way, the pH was adjusted to 6.5 with 1 mol/L NaOH solution, and it was kept for 10 min at 37 °C. Then, added 0.125 g trypsin and 1.25 g bile, mixed well. The enteric II stage was digested for 2 h at 37 °C for 150 rpm in a water bath oscillator in the dark condition. After digestion, it was heated to boiling in water for 2 min and stored.
The stored samples of each digestion stage were centrifuged at 12,000 rpm for 5 min at 4 °C. The supernatant was collected and used to determine chemical composition. The rest residue was dried at 60 °C and used for SEM.
Bioaccessibility was considered as the concentration of bioactive compounds released from the food matrix by in vitro enteric digestion. The bioaccessibility is calculated according to the following equation:
where BCdigested and BCnon-digested correspond to the bioactive compound concentration in digested and non-digested Radix Astragali, respectively.
Five g sample was weighted accurately and 100 mL of 70% ethanol was added to a conical flask. The mixture was extracted by ultrasound bath for 60 min with the power of 100 W. The extracted samples were filtered and centrifuged at 12,000 rpm for 5 min at 4 °C. The supernatant was collected and used to determine chemical composition.
The total flavonoid content was determined following methods by Liu, et al.17, and slightly modified. Rutin was used as a standard for the calibration curve. Rutin was dissolved in ethanol with an initial concentration of 0.2 mg/mL. The different volumes of the stock solution with 0, 0.2, 0.4, 0.8, 1.2, 1.6, 2.0 and 2.4 mL were transferred into 10 mL volumetric flasks and were added 2 mL 70% ethanol. Shaken evenly, 0.3 mL of 5% NaNO2 solution was added and hold for 6 min. Then, 0.3 mL of 10% AlCl3 solution was added and the mixture was shaken evenly and hold for 6 min. After that, 4 mL of the 4% NaOH solution was added in the volumetric flask. The mixture was fixed volume to scale with 70% ethanol and hold for 15 min. The absorbance of these mixtures was measured at 510 nm using TU-1810 APC UV–Visible spectrophotometer (Beijing Persee General Instrument Co., Ltd, Beijing, China) and the standard curve was established.
Three millilitre of sample solution prepared above were transferred in 10 mL volumetric flasks. The absorbency of the sample was determined by the colorimetric method as described above.
The total saponins content was determined following methods by18, and slightly modified. AG IV was used as a standard for the calibration curve. AG IV was dissolved in ethanol with an initial concentration of 0.5 mg/mL. The different volumes of the stock solution with 0.00, 0.05, 0.10, 0.20 0.30, 0.40 mL were transferred into 10 mL volumetric flasks and were added 70% ethanol up to 0.5 mL. Shaken evenly, 0.5 mL of 8% vanillin ethanol solution and 5.0 mL of 72% sulfuric acid were added. After mixing evenly, the reaction mixture was incubated at 62 ℃ for 20 min. Then, the mixture was cooled in an ice water bath to room temperature. The absorbance of these mixtures was measured at 540 nm using TU-1810 APC UV–Visible spectrophotometer and the standard curve was established.
Sample solution (0.5 mL) prepared above were transferred in 10 mL volumetric flasks. The absorbency of the sample was determined by the colorimetric method as described above.
Astragalus flavonoids were analyzed by HPLC, using a Thermo Scientific Dionex Ultimate 3000 series HPLC System (Dionex, USA), diode array detector (Dionex, USA) and a Kromasil 100–5-C18 column (4.6 by 250 mm). Column temperature was maintained at 30 °C with 10 µL injection volume. The mobile phase consisted of 0.05% formic acid in water (A) and acetonitrile (B) using the following gradient elution sequence: 0–5 min, 0–5% B; 5–10 min, 5–20% B; 10–25 min, 20–25% B; 25–35 min, 25–30% B; 35–40 min, 30–35% B; 40–54 min, 35–40% B; 54–55 min, 40–0% B. The flow rate was set at 1 mL/min. Flavonoids were detected and quantified by monitoring the absorbance at 254 nm, including calycosin-glu, genistin, Ononin, calycosin and formononetin, their molecular formulae and chemical structures were shown in Table 5. The concentrations of different analytes were determined according to the corresponding standard curves. The regression equations were y1 = 640.7x−0.1856 (R2 = 0.9994, n = 6); y2 = 886.38x−0.2805 (R2 = 0.9995, n = 6); y3 = 746.76x−0.2932 (R2 = 0.9995, n = 6); y4 = 995.48x−0.383 (R2 = 0.9995, n = 6); y5 = 1234x−0.5045 (R2 = 0.9994, n = 6), where y was the peak area of analyte, and x was the concentration of analyte (mg/mL).
A DGU-20A3R HPLC system (SHIMADZU, Japan) coupled with an ELSD-16 (SHIMADZU, Japan) was used for the determination of the AG IV and AG II, whose molecular formulae and chemical structures were shown in Table 5. The chromatographic separations were performed on a Phenomenex C18 column (4.60 × 250 mm, 5 μm) maintained at room temperature. The mobile phases consisted of acetonitrile (A) and water (B) with a gradient elution as follows: 0–20 min, 96–80% B; 20–40 min, 80–70% B; 40–65 min, 70–40% B; 65–70 min, 40–96% B; 70–80 min, 96% B. A constant flow rate of 1.0 mL/min was employed. The drift tube temperature of the ELSD was set at 100 °C, and the carrier gas flow rate was 2.5 L/min. The sample injection volume was 20 μL. The regression equations for AG IV and AG II were y6 = 1.5346x + 0.3752 (R2 = 0.9980, n = 6); y7 = 1.644x + 0.1816 (R2 = 1.0000, n = 6), where y was the peak area of analyte, and x was the concentration of analyte (mg/mL).
A EVO LS15 scanning electron microscope (Zeiss, Germany) with Q150RS ion sputtering coater (Quorum, UK) were used to examine the morphological alterations of samples. The samples of before and after fermentation and the sample residue from each stage of in vitro digestion were dried at 60 °C and crushed. The crushed samples were fixed on the SEM sample stage with conductive adhesive and were coated with gold on the sample surface using an ion sputter coater. Then, the samples were observed at an accelerating voltage of 10 kv (2000× magnification).
The supernatant prepared above were concentrated to dryness in RE-52AA rotary evaporator device (Shanghai Yarong Biochemical Instrument Factory, Shanghai, China) under vacuum at 55 °C. The dried extract was dissolved by anhydrous ethanol to prepared different concentrations sample solution for the determination of antioxidant activity.
The antioxidant activity was performed through 2,2-diphenyl-1-picrylhydrazyl (DPPH) free-radical scavenging activity. The antioxidant activity was determined according to the method of Amarowicz, et al.19 and Chen, et al.20, with some modification. One mg/mL sample solution 100 μL was mixed with 1 mL of 0.08 mg/mL DPPH ethanol solution. Then, anhydrous ethanol was added to 4 mL. The mixture was shaken vigorously and incubated in darkness for 30 min. The absorbance of the solution was measured at 517 nm using TU-1810 APC UV–Visible spectrophotometer. Vc was used as a reference compound. All tests were carried out in triplicate. The free radical scavenging activity is calculated according to the following equation:
where AB is the absorbance of the control (DPPH without sample), AA is the absorbance of the reaction mixture.
The antioxidant activity was performed through 2,2-hydrazine bis (3-ethylbenzothiazoline-6-sulfonic acid) diamine salt radical cation (ABTS+) scavenging activity. The antioxidant activity was determined according to the method of Ali et al.21, with some modification. Four millilitre of ABTS solution was added to 0.1 mL of various concentrations of the sample solution. The mixture was shaken vigorously and incubated in darkness for 6 min. The absorbance of the solution was measured at 734 nm using TU-1810 APC UV–Visible spectrophotometer. Vc was used as reference compound. The free radical scavenging activity is calculated according to the following equation :
where AB is the absorbance of the control (ABTS without sample), AA is the absorbance of the reaction mixture.
Statistics were done using SPSS 26 software. All the data were presented as mean ± standard error (SE) from three independent parallel experiments. Significant differences among the means of samples were analyzed by Duncan’s test with a 95% confidence level14. Origin 2018 software was used to graph the results.
The datasets used and/or analysed during the current study are available from the first author Cai-Yun Chen on reasonable request.
Fan, Y. et al. Effects of Astragalus polysaccharide liposome on lymphocyte proliferation in vitro and adjuvanticity in vivo. Carbohyd. Polym. 88, 68–74. https://doi.org/10.1016/j.carbpol.2011.11.067 (2012).
Li, M. et al. Biological active ingredients of Astragali Radix and its mechanisms in treating cardiovascular and cerebrovascular diseases. Phytomedicine 98, 153918. https://doi.org/10.1016/j.phymed.2021.153918 (2022).
Article CAS PubMed Google Scholar
Ren , S. , H. Zhang , Y. Mu , M. Sun & Liu , P. Pharmacological effects of astragaloside IV: A literature review .J. Tradit.Chin.Med.15 , 413–416 .https://doi.org/10.1016/S0254-6272(13)60189-2 (2013).
Li, L., Hou, X., Xu, R., Liu, C. & Tu, M. Research review on the pharmacological effects of astragaloside IV. Fundam. Clin. Pharmacol. 31, 17–36. https://doi.org/10.1111/fcp.12232 (2017).
Article CAS PubMed Google Scholar
Yao, M.-L. et al. A novel biotransformation of astragalosides to astragaloside IV with the deacetylation of fungal endophyte Penicillium canescens. Process Biochem. 49, 807–812. https://doi.org/10.1016/j.procbio.2014.02.014 (2014).
Wu, J. G. et al. Biotransformation of vine tea (Ampelopsis grossedentata) by solid-state fermentation using medicinal fungus Poria cocos. J. Food Sci. Technol.-Mysore 53, 3225–3232. https://doi.org/10.1007/s13197-016-2297-6 (2016).
Tu, Y. et al. Extracts of Poria cocos improve functional dyspepsia via regulating brain-gut peptides, immunity and repairing of gastrointestinal mucosa. Phytomedicine 95, 153875. https://doi.org/10.1016/j.phymed.2021.153875 (2022).
Article CAS PubMed Google Scholar
Cheng, Y. et al. Structural characterization and hepatoprotective activity of a galactoglucan from Poria cocos. Carbohydr. Polym. 263, 117979. https://doi.org/10.1016/j.carbpol.2021.117979 (2021).
Article CAS PubMed Google Scholar
Lu, M.-K., Chao, C.-H. & Hsu, Y.-C. Effect of carbohydrate-feeding strategy on the production, physiochemical changes, anti-inflammation activities of polysaccharides of Poria cocos. Int. J. Biol. Macromol. 192, 435–443. https://doi.org/10.1016/j.ijbiomac.2021.10.017 (2021).
Article CAS PubMed Google Scholar
Huynh, N., Van Camp, J., Smagghe, G. & Raes, K. Improved release and metabolism of flavonoids by steered fermentation processes: A review. Int. J. Mol. Sci. 15, 19369–19388. https://doi.org/10.3390/ijms151119369 (2014).
Article CAS PubMed Google Scholar
He, et al. Recent advances in biotransformation of saponins. Molecules 24, 2365. https://doi.org/10.3390/molecules24132365 (2019).
Article CAS PubMed PubMed Central Google Scholar
Shen, D. et al. Preparation and aroma analysis of flavonoid-rich ginkgo seeds fermented using rice wine starter. Food Biosci. 44, 101459. https://doi.org/10.1016/j.fbio.2021.101459 (2021).
Liu, Z. et al. Diagnostic product ions-based chemical characterization and antioxidative activity evaluation of solid fermentation for Astragali radix produced by Paecilomyces cicadae. Arab. J. Chem. 14, 102908. https://doi.org/10.1016/j.arabjc.2020.11.014 (2021).
Ningtyas, D. W., Hati, S. & Prakash, S. Bioconversion and bioaccessibility of isoflavones from sogurt during in vitro digestion. Food Chem. 343, 128553. https://doi.org/10.1016/j.foodchem.2020.128553 (2021).
Article CAS PubMed Google Scholar
Gullon , B. , Pintado , ME , Fernandez-Lopez , J. , Perez-Alvarez , JA & Widow-Martos , M. In vitro gastrointestinal digestion of pomegranate peel (Punica granatum) flour obtained from co-products: Changes in the antioxidant potential and stability of bioactive compounds.J. Funct.Foods 19, 617–628.https://doi.org/10.1016/j.jff.2015.09.056 (2015).
Larkin, T., Price, W. E. & Astheimer, L. The key importance of soy isoflavone bioavailability to understanding health benefits. Crit. Rev. Food Sci. Nutr. 48, 538–552. https://doi.org/10.1080/10408390701542716 (2008).
Article CAS PubMed Google Scholar
Liu, X. M. et al. Effects of five extraction methods on total content, composition, and stability of flavonoids in jujube. Food Chem. X 14, 100287. https://doi.org/10.1016/j.fochx.2022.100287 (2022).
Article CAS PubMed PubMed Central Google Scholar
Liu, Y. et al. Quantitative analysis and screening for key genes related to tea saponin in Camellia oleifera Abel. seeds. Food Biosci. 49, 101901. https://doi.org/10.1016/j.fbio.2022.101901 (2022).
Amarowicz, R., Pegg, R. B., Rahimi-Moghaddam, P., Barl, B. & Weil, J. A. Free-radical scavenging capacity and antioxidant activity of selected plant species from the Canadian prairies. Food Chem. 84, 551–562. https://doi.org/10.1016/s0308-8146(03)00278-4 (2004).
Chen, C. Y. et al. Simultaneous separation of glycyrrhizic acid, baicalein and wogonin from Radix Glycyrrhizae and Radix Scutellariae using foam fractionation and in vitro activity evaluation. J. Sci. Food Agric. 102, 5200–5209. https://doi.org/10.1002/jsfa.11872 (2022).
Article CAS PubMed Google Scholar
Ali, I. et al. Potent α-amylase inhibitors and radical (DPPH and ABTS) scavengers based on benzofuran-2-yl(phenyl)methanone derivatives: Syntheses, in vitro, kinetics, and in silico studies. Bioorg. Chem. 104, 104238. https://doi.org/10.1016/j.bioorg.2020.104238 (2020).
Article CAS PubMed Google Scholar
This research was financially supported by General Program of National Natural Science Foundation of China (82174039), General project of Shandong Natural Science Foundation (ZR2020MH371), Shandong Taishan Scholars Young Expert Project (tsqn202103110), Young and Creative Team for Talent Introduction of Shandong Province (10073004), Major Scientific and Technological Innovation Projects in Shandong Province (2021CXGC010511), Traditional Chinese Medicine Planning Project of Shandong Province (2021M139), Binzhou Social Science Planning Project (23-SKGH-002). Ministry of Education Industry-University-Research Collaboration education project (220500524270338).
These authors contributed equally: Cai-Yun Chen and Run Zhang.
School of Public Health and Management, Binzhou Medical University, Yantai, People’s Republic of China
Cai-Yun Chen, Li-Jie Zhang, Zhi-Yong Hu, Xue Mei & Wei Mi
School of Pharmaceutical Science, Binzhou Medical University, Yantai, 264003, People’s Republic of China
Run Zhang, Shao-Ping Wang & Jia-Yu Zhang
You can also search for this author in PubMed Google Scholar
You can also search for this author in PubMed Google Scholar
You can also search for this author in PubMed Google Scholar
You can also search for this author in PubMed Google Scholar
You can also search for this author in PubMed Google Scholar
You can also search for this author in PubMed Google Scholar
You can also search for this author in PubMed Google Scholar
You can also search for this author in PubMed Google Scholar
R.Z. and C.C wrote original draft and methodology. Z.H. and S.W wrote review and editing. X.M. and L.Z helped calculate and analyze data. J. Z. and W.M supervision and funding acquisition. All authors reviewed the manuscript and agreed to the published version of the manuscript.
Correspondence to Wei Mi or Jia-Yu Zhang.
The authors declare no competing interests.
Springer Nature remains neutral with regard to jurisdictional claims in published maps and institutional affiliations.
Open Access This article is licensed under a Creative Commons Attribution 4.0 International License, which permits use, sharing, adaptation, distribution and reproduction in any medium or format, as long as you give appropriate credit to the original author(s) and the source, provide a link to the Creative Commons licence, and indicate if changes were made. The images or other third party material in this article are included in the article's Creative Commons licence, unless indicated otherwise in a credit line to the material. If material is not included in the article's Creative Commons licence and your intended use is not permitted by statutory regulation or exceeds the permitted use, you will need to obtain permission directly from the copyright holder. To view a copy of this licence, visit http://creativecommons.org/licenses/by/4.0/.
Chen, CY., Zhang, R., Zhang, LJ. et al. Biotransformation and bioaccessibility of active ingredients from Radix Astragali by Poria cocos during solid-state fermentation and in vitro digestion and antioxidant activity evaluation. Sci Rep 13, 6888 (2023). https://doi.org/10.1038/s41598-023-33969-4
DOI: https://doi.org/10.1038/s41598-023-33969-4
Anyone you share the following link with will be able to read this content:
Sorry, a shareable link is not currently available for this article.
Provided by the Springer Nature SharedIt content-sharing initiative
By submitting a comment you agree to abide by our Terms and Community Guidelines. If you find something abusive or that does not comply with our terms or guidelines please flag it as inappropriate.
Scientific Reports (Sci Rep) ISSN 2045-2322 (online)
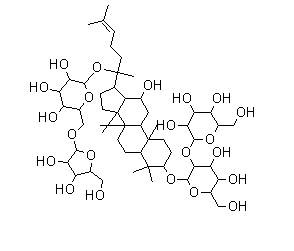
Magnolin Sign up for the Nature Briefing: Translational Research newsletter — top stories in biotechnology, drug discovery and pharma.